Bioengineered materials with selective antimicrobial toxicity in ... - Military Medical Research
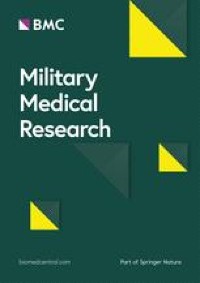
Hernando-Amado S, Coque TM, Baquero F, Martínez JL. Defining and combating antibiotic resistance from one health and global health perspectives. Nat Microbiol. 2019;4(9):1432–42.
Ellabaan MMH, Munck C, Porse A, Imamovic L, Sommer MOA. Forecasting the dissemination of antibiotic resistance genes across bacterial genomes. Nat Commun. 2021;12(1):2435.
Zafar H, Yousefiasl S, Raza F. T-cell membrane-functionalized nanosystems for viral infectious diseases. Mater Chem Horizons. 2023;2(1):41–8.
Abedon ST. Bacterial 'immunity' against bacteriophages. Bacteriophage. 2012;2(1):50–4.
Jamaledin R, Yiu CKY, Zare EN, Niu LN, Vecchione R, Chen G, et al. Advances in antimicrobial microneedle patches for combating infections. Adv Mater. 2020;32(33):e2002129.
Wang CY, Makvandi P, Zare EN, Tay FR, Niu LN. Advances in antimicrobial organic and inorganic nanocompounds in biomedicine. Adv Ther. 2020;3(8):2000024.
Li W, Thian ES, Wang M, Wang Z, Ren L. Surface design for antibacterial materials: from fundamentals to advanced strategies. Adv Sci. 2021;8(19):e2100368.
Zhang X, Chen X, Yang J, Jia HR, Li YH, Chen Z, et al. Quaternized silicon nanoparticles with polarity-sensitive fluorescence for selectively imaging and killing Gram-positive bacteria. Adv Funct Mater. 2016;26(33):5958–70.
Slavin YN, Asnis J, Häfeli UO, Bach H. Metal nanoparticles: understanding the mechanisms behind antibacterial activity. J Nanobiotechnology. 2017;15(1):65.
Makvandi P, Wang C, Zare EN, Borzacchiello A, Niu L, Tay FR. Metal-based nanomaterials in biomedical applications: antimicrobial activity and cytotoxicity aspects. Adv Funct Mater. 2020;30(22):1910021.
Arts IS, Gennaris A, Collet JF. Reducing systems protecting the bacterial cell envelope from oxidative damage. FEBS Lett. 2015;589(14):1559–68.
Bertani B, Ruiz N. Function and biogenesis of lipopolysaccharides. EcoSal Plus. 2018;8(1):25. https://doi.org/10.1128/ecosalplus.ESP-0001-2018.
Lerouge I, Vanderleyden J. O-antigen structural variation: mechanisms and possible roles in animal/plant-microbe interactions. FEMS Microbiol Rev. 2022;26(1):17–47.
Ranava D, Caumont-Sarcos A, Albenne C, Ieva R. Bacterial machineries for the assembly of membrane-embedded β-barrel proteins. FEMS Microbiol Lett. 2018. https://doi.org/10.1093/femsle/fny087.
Konovalova A, Kahne DE, Silhavy TJ. Outer membrane biogenesis. Annu Rev Microbiol. 2017;71:539–56.
Ghai I, Ghai S. Understanding antibiotic resistance via outer membrane permeability. Infect Drug Resist. 2018;11:523–30.
Gupta A, Mumtaz S, Li CH, Hussain I, Rotello VM. Combatting antibiotic-resistant bacteria using nanomaterials. Chem Soc Rev. 2019;48(2):415–27.
Benfield AH, Henriques ST. Mode-of-action of antimicrobial peptides: membrane disruption vs. intracellular mechanisms. Front Med Technol. 2020;2:610997.
Porfírio S, Carlson RW, Azadi P. Elucidating peptidoglycan structure: an analytical toolset. Trends Microbiol. 2019;27(7):607–22.
Rohde M. The Gram-positive bacterial cell wall. Microbiol Spectr. 2019. https://doi.org/10.1128/microbiolspec.GPP3-0044-2018.
Rajagopal M, Walker S. Envelope structures of Gram-positive bacteria. Curr Top Microbiol Immunol. 2017;404:1–44.
Malanovic N, Lohner K. Gram-positive bacterial cell envelopes: the impact on the activity of antimicrobial peptides. Biochim Biophys Acta. 2016;1858(5):936–46.
Doyle L, Ovchinnikova OG, Myler K, Mallette E, Huang B, Lowary T, et al. Biosynthesis of a conserved glycolipid anchor for Gram-negative bacterial capsules. Nat Chem Biol. 2019;15(6):632–40.
Angelin J, Kavitha M. Exopolysaccharides from probiotic bacteria and their health potential. Int J Biol Macromol. 2020;62:853–65.
Gow NAR, Latge JP, Munro CA. The fungal cell wall: structure, biosynthesis, and function. Microbiol Spectr. 2017. https://doi.org/10.1128/microbiolspec.FUNK-0035-2016.
Garcia-Rubio R, de Oliveira HC, Rivera J, Trevijano-Contador N. The fungal cell wall: candida, cryptococcus, and aspergillus species. Front Microbiol. 2020;10:2993.
Hasim S, Coleman JJ. Targeting the fungal cell wall: current therapies and implications for development of alternative antifungal agents. Future Med Chem. 2019;11(8):869–83.
Wang Y, Yang Y, Shi Y, Song H, Yu C. Antibiotic-free antibacterial strategies enabled by nanomaterials: progress and perspectives. Adv Mater. 2020;32(18):e1904106.
Valencia C, Martínez-Castañón GA, Martínez-Martínez RE, Loyola-Rodríguez JP, Reyes-Macías JF, Ora-Zarzosa G, et al. Bactericide efficiency of a combination of chitosan gel with silver nanoparticles. Mater Lett. 2013;106:413–6.
Antonelli M, De Pascale G, Ranier VM, Guaglianone E, Donellih G. Comparison of triple-lumen central venous catheters impregnated with silver nanoparticles (AgTive®) vs conventional catheters in intensive care unit patients. J Hosp Infect. 2012;82(2):101–7.
Ehsan Z, Denise Wetzel J. John P Clancy BS, Nebulized liposomal amikacin for the treatment of pseudomonas aeruginosa infection in cystic fibrosis patients. Expert Opin Investig Drugs. 2014;23(5):743–9.
Chandel NS, Scott Budinger GR. The good and the bad of antibiotics. Sci Transl Med. 2013;5(192):192fs25.
Masri A, Anwar A, Khan NA, Siddiqui R. The use of nanomedicine for targeted therapy against bacterial infections. Antibiotics. 2019;8(4):260.
Matsuzaki K. Control of cell selectivity of antimicrobial peptides. Biochim Biophys Acta. 2009;1788(8):1687–92.
Bobone S, Stella L. Selectivity of antimicrobial peptides: a complex interplay of multiple equilibria. Adv Exp Med Biol. 2019;1117:175–214.
Moran GJ, Krishnadasan A, Gorwitz RJ, Fosheim GE, McDougal LK, Carey RB, et al. Methicillin-resistant S. aureus infections among patients in the emergency department. N Engl J Med. 2006;355(7):666–74.
Lehar SM, Pillow T, Xu M, Staben L, Kajihara KK, Vandlen R, et al. Novel antibody-antibiotic conjugate eliminates intracellular S. aureus. Nature. 2015;527(7578):323–8.
Scudiero O, Brancaccio M, Mennitti C, Laneri S, Lombardo B, De Biasi MG, et al. Human defensins: a novel approach in the fight against skin colonizing Staphylococcus aureus. Antibiotics. 2020;9(4):198.
Hussain S, Joo J, Kang J, Kim B, Braun GB, She ZG, et al. Antibiotic-loaded nanoparticles targeted to the site of infection enhance antibacterial efficacy. Nat Biomed Eng. 2018;2(2):95–103.
Yang S, Han X, Yang Y, Qiao H, Yu Z, Liu Y, et al. Bacteria-targeting nanoparticles with microenvironment-responsive antibiotic release to eliminate intracellular Staphylococcus aureus and associated infection. ACS Appl Mater Interfaces. 2018;10(17):14299–311.
Barna JC, Williams DH. The structure and mode of action of glycopeptide antibiotics of the vancomycin group. Annu Rev Microbiol. 1984;38:339–57.
Wang H, Song Z, Li S, Wu Y, Han H. One stone with two birds: functional gold nanostar for targeted combination therapy of drug-resistant Staphylococcus aureus infection. ACS Appl Mater Interfaces. 2019;11(36):32659–69.
Vukomanović M, Žunič V, Kunej Š, Jančar B, Jeverica S, Podlipec R, et al. Nano-engineering the antimicrobial spectrum of lantibiotics: activity of nisin against Gram negative bacteria. Sci Rep. 2017;7(1):4324.
Yuan K, Jurado-Sánchez B, Escarpa A. Dual-propelled lanbiotic based janus micromotors for selective inactivation of bacterial biofilms. Angew Chem Int Ed Engl. 2021;60(9):4915–24.
Lee H, Lim SI, Shin SH, Lim Y, Koh JW, Yang S. Conjugation of cell-penetrating peptides to antimicrobial peptides enhances antibacterial activity. ACS Omega. 2019;4(13):15694–701.
Li J, Shang L, Lan J, Chou S, Feng X, Shi B, et al. Targeted and intracellular antibacterial activity against S. agalactiae of the chimeric peptides based on pheromone and cell-penetrating peptides. ACS Appl Mater Interfaces. 2020;12(40):44459–74.
Brezden A, Mohamed MF, Nepal M, Harwood JS, Kuriakose J, Seleem MN, et al. Dual targeting of intracellular pathogenic bacteria with a cleavable conjugate of kanamycin and an antibacterial cell-penetrating peptide. J Am Chem Soc. 2016;138(34):10945–9.
Dabbagh Moghaddam F, Romana BF. Application of microfluidic platforms in cancer therapy. Mater Chem Horizons. 2022;1(1):69–88.
Raza F, Zafar H, Khan AU, Hatami K. T-cell membrane-coated nanomaterials in cancer treatment. Mater Chem Horizons. 2022;1(3):199–217.
Zurawski DV, McLendon MK. Monoclonal antibodies as an antibacterial approach against bacterial pathogens. Antibiotics. 2020;9(4):155.
Le H, Arnoult C, Dé E, Schapman D, Galas L, Le Cerf D, et al. Antibody-conjugated nanocarriers for targeted antibiotic delivery: application in the treatment of bacterial biofilms. Biomacromol. 2021;22(4):1639–53.
Alhmoud H, Cifuentes-Rius A, Delalat B, Lancaster DG, Voelcker NH. Gold-decorated porous silicon nanopillars for targeted hyperthermal treatment of bacterial infections. ACS Appl Mater Interfaces. 2017;9(39):33707–16.
Yan S, Chen S, Gou X, Yang J, An J, Jin X, et al. Biodegradable supramolecular materials based on cationic polyaspartamides and pillar[5]arene for targeting Gram-positive bacteria and mitigating antimicrobial resistance. Adv Funct Mater. 2019;29(38):1904683.
Brives C, Pourraz J. Phage therapy as a potential solution in the fight against AMR: obstacles and possible futures. Palgrave Commun. 2020;6:100.
Onsea J, Soentjens P, Djebara S, Merabishvili M, Depypere M, Spriet I, et al. Bacteriophage application for difficult-to-treat musculoskeletal infections: development of a standardized multidisciplinary treatment protocol. Viruses. 2019;11(10):891.
Ul Haq I, Chaudhry WN, Akhtar MN, Andleeb S, Qadri I. Bacteriophages and their implications on future biotechnology: a review. Virol J. 2012;9:9.
Dunne M, Hupfeld M, Klumpp J, Loessner MJ. Molecular basis of bacterial host interactions by Gram-positive targeting bacteriophages. Viruses. 2018;10(8):397.
Hopf J, Waters M, Kalwajtys V, Carothers KE, Roeder RK, Shrout JD, et al. Phage-mimicking antibacterial core-shell nanoparticles. Nanoscale Adv. 2019;1(12):4812–26.
Hathaway H, Ajuebor J, Stephens L, Coffey A, Potter U, Sutton JM, et al. Thermally triggered release of the bacteriophage endolysin CHAPK and the bacteriocin lysostaphin for the control of methicillin resistant Staphylococcus aureus (MRSA). J Control Release. 2017;245:108–15.
Dik DA, Fisher JF, Mobashery S. Cell-wall recycling of the Gram-negative bacteria and the nexus to antibiotic resistance. Chem Rev. 2018;118(12):5952–84.
Lam SJ, O'Brien-Simpson NM, Pantarat N, Sulistio A, Wong EHH, Chen YY, et al. Combating multidrug-resistant Gram-negative bacteria with structurally nanoengineered antimicrobial peptide polymers. Nat Microbiol. 2016;1(11):16162.
Seefeldt AC, Nguyen F, Antunes S, Pérébaskine N, Graf M, Arenz S, et al. The proline-rich antimicrobial peptide Onc112 inhibits translation by blocking and destabilizing the initiation complex. Nat Struct Mol Biol. 2015;22(6):470–5.
Hu X, Huang YY, Wang Y, Wang X, Hamblin MR. Antimicrobial photodynamic therapy to control clinically relevant biofilm infections. Front Microbiol. 2018;9:1299.
Wang W, Hao C, Sun M, Xu L, Wu X, Xu C, et al. Peptide mediated chiral inorganic nanomaterials for combating Gram-negative bacteria. Adv Funct Mater. 2018;28(44):1805112.
Liu F, Ni ASY, Lim Y, Mohanram H, Bhattacharjya S, Xing B. Lipopolysaccharide neutralizing peptide-porphyrin conjugates for effective photoinactivation and intracellular imaging of Gram-negative bacteria strains. Bioconjug Chem. 2012;23(8):1639–47.
Ebbensgaard A, Mordhorst H, Aarestrup FM, Hansen EB. The role of outer membrane proteins and lipopolysaccharides for the sensitivity of Escherichia coli to antimicrobial peptides. Front Microbiol. 2018;9:2153.
Cantelli A, Piro F, Pecchini P, Di Giosia M, Danielli A, Calvaresi M. Concanavalin A-Rose Bengal bioconjugate for targeted Gram-negative antimicrobial photodynamic therapy. J Photochem Photobiol B. 2020;206:111852.
Chen J, Li X, Li L, Zhang T, Zhang Q, Wu F, et al. Coagulation factors VII, IX and X are effective antibacterial proteins against drug-resistant Gram-negative bacteria. Cell Res. 2019;29(9):711–24.
Ruehle B, Clemens DL, Lee BY, Horwitz MA, Zink JI. A pathogen-specific cargo delivery platform based on mesoporous silica nanoparticles. J Am Chem Soc. 2017;139(19):6663–8.
Storek KM, Auerbach MR, Shi H, Garcia NK, Sun D, Nickerson NN, et al. Monoclonal antibody targeting the β-barrel assembly machine of Escherichia coli is bactericidal. Proc Natl Acad Sci U S A. 2018;115(14):3692–7.
Jault P, Leclerc T, Jennes S, Pirnay JP, Que YA, Resch G, et al. Efficacy and tolerability of a cocktail of bacteriophages to treat burn wounds infected by Pseudomonas aeruginosa (PhagoBurn): a randomised, controlled, double-blind phase 1/2 trial. Lancet Infect Dis. 2019;19(1):35–45.
He X, Yang Y, Guo Y, Lu S, Du Y, Li JJ, et al. Phage-guided targeting, discriminative imaging, and synergistic killing of bacteria by AIE bioconjugates. J Am Chem Soc. 2020;142(8):3959–69.
Peng H, Borg RE, Dow LP, Pruitt BL, Chen IA. Controlled phage therapy by photothermal ablation of specific bacterial species using gold nanorods targeted by chimeric phages. Proc Natl Acad Sci USA. 2020;117(4):1951–61.
Krom RJ, Bhargava P, Lobritz MA, Collins JJ. Engineered phagemids for nonlytic, targeted antibacterial therapies. Nano Lett. 2015;15(7):4808–13.
Huma ZE, Gupta A, Javed I, Das R, Hussain SZ, Mumtaz S, et al. Cationic silver nanoclusters as potent antimicrobials against multidrug-resistant bacteria. ACS Omega. 2018;3(12):16721–7.
Kim D, Kwon SJ, Wu X, Sauve J, Lee I, Nam J, et al. Selective killing of pathogenic bacteria by antimicrobial silver nanoparticle-cell wall binding domain conjugates. ACS Appl Mater Interfaces. 2018;10(16):13317–24.
Tsuchido Y, Horiuchi R, Hashimoto T, Ishihara K, Kanzawa N, Hayashita T. Rapid and selective discrimination of Gram-positive and Gram-negative bacteria by boronic acid-modified poly(amidoamine) dendrimer. Anal Chem. 2019;91(6):3929–35.
Luk BT, Zhang L. Cell membrane-camouflaged nanoparticles for drug delivery. J Control Release. 2015;220(Pt B):600–7.
Fang RH, Kroll AV, Gao W, Zhang L. Cell membrane coating nanotechnology. Adv Mater. 2018;30(23):e1706759.
Wu S, Huang Y, Yan J, Li Y, Wang J, Yang YY, et al. Bacterial outer membrane-coated mesoporous silica nanoparticles for targeted delivery of antibiotic rifampicin against Gram-negative bacterial infection in vivo. Adv Funct Mater. 2021;31(35):2103442.
Zhu N, Zhong C, Liu T, Zhu Y, Gou S, Bao H, et al. Newly designed antimicrobial peptides with potent bioactivity and enhanced cell selectivity prevent and reverse rifampin resistance in Gram-negative bacteria. Eur J Pharm Sci. 2021;158:105665.
Sartorio MG, Pardue EJ, Feldman MF, Haurat MF. Bacterial outer membrane vesicles: from discovery to applications. Annu Rev Microbiol. 2021;75:609–30.
Ikeh M, Ahmed Y, Quinn J. Phosphate acquisition and virulence in human fungal pathogens. Microorganisms. 2017;5(3):48.
Ibe C, Munro CA. Fungal cell wall: an underexploited target for antifungal therapies. PLoS Pathog. 2021;17(4):e1009470.
Campoy S, Adrio JL. Antifungals. Biochem Pharmacol. 2017;133:86–96.
Voltan AR, Quindós G, Alarcón KPM, Fusco-Almeida AM, Mendes-Giannini MJS, Chorilli M. Fungal diseases: could nanostructured drug delivery systems be a novel paradigm for therapy? Int J Nanomedicine. 2016;11:3715–30.
Moen MD, Lyseng-Williamson KA, Scott LJ. Liposomal amphotericin B: a review of its use as empirical therapy in febrile neutropenia and in the treatment of invasive fungal infections. Drugs. 2009;69(3):361–92.
Ambati S, Ferarro AR, Kang SE, Lin J, Lin X, Momany M, et al. Dectin-1-targeted antifungal liposomes exhibit enhanced efficacy. Sphere. 2019;4(1):e00025-19.
McLellan CA, Vincent BM, Solis NV, Lancaster AK, Sullivan LB, Hartland CL, et al. Inhibiting mitochondrial phosphate transport as an unexploited antifungal strategy. Nat Chem Biol. 2018;14(12):135–41.
Fedorova O, Jagdmann GE, Adams RL, Yuan L, Van Zandt MC, Pyle AM. Small molecules that target group II introns are potent antifungal agents. Nat Chem Biol. 2018;14(12):1073–8.
Wang X, Wang A, Qiu L, Chen M, Lu A, Li G, et al. Expedient discovery for novel antifungal leads targeting succinate dehydrogenase: pyrazole-4-formylhydrazide derivatives bearing a diphenyl ether fragment. J Agric Food Chem. 2020;68(49):14426–37.
Wu YY, Bin SW, Zhu JJ, Long ZQ, Liu LW, Wang PY, et al. Novel 1,3,4-oxadiazole-2-carbohydrazides as prospective agricultural antifungal agents potentially targeting succinate dehydrogenase. ...
Comments
Post a Comment